Melatonin, an ancient ally against pancreatic disorders
Melatonin and pancreas
Abstract
Melatonin is mainly produced in the pineal gland of mammals with a circadian rhythm. It has also been synthesized in different organs and tissues including the gastrointestinal tract. Additionally, melatonin is widely present in plants and foodstuff. In addition to its effects on the sleep-wake cycle and reproductive regulation in photoperiodic animals, melatonin regulates a wide variety of cellular processes, participating in the control of antioxidant defenses, immune response, energy metabolism, cell growth and proliferation, having beneficial effects on most of the tissues and organs, including the pancreas.
Here, we have reviewed the recent findings related to the effects of melatonin on the physiology of the exocrine pancreas. Of major relevance, the effects of the indoleamine on pathological processes such as cancer, inflammation, diabetes, and fibrosis are also reviewed. Not less important, its effects on normal/healthy cells of the pancreas to modulate normal physiological functions are discussed.
References
2. Manchester LC, Coto-Montes A, Boga JA, et al. (2015) Melatonin: an ancient molecule that makes oxygen metabolically tolerable. J. Pineal Res. 59: 403–419. https://doi.org/10.1111/jpi.12267.
3. Amaral FG do, Cipolla-Neto J (2018) A brief review about melatonin, a pineal hormone. Arch. Endocrinol. Metab. 62: 472–479. https://doi.org/10.20945/2359-3997000000066.
4. Zhao D, Yu Y, Shen Y, et al. (2019) Melatonin synthesis and function: Evolutionary history in animals and plants. Front. Endocrinol. (Lausanne) 10: 249. https://doi.org/10.3389/fendo.2019.00249.
5. Tan D-X, Manchester LC, Liu X, et al. (2013) Mitochondria and chloroplasts as the original sites of melatonin synthesis: a hypothesis related to melatonin’s primary function and evolution in eukaryotes. J. Pineal Res. 54: 127–138. https://doi.org/10.1111/jpi.12026.
6. Ostrin LA (2019) Ocular and systemic melatonin and the influence of light exposure. Clin. Exp. Optom. 102: 99–108. https://doi.org/10.1111/cxo.12824.
7. Acuña-Castroviejo D, Escames G, Venegas C, et al. (2014) Extrapineal melatonin: sources, regulation, and potential functions. Cell Mol. Life Sci. 71: 2997–3025. https://doi.org/10.1007/s00018-014-1579-2.
8. Cheng G, Ma T, Deng Z, et al. (2021) Plant-derived melatonin from food: a gift of nature. Food Funct. 12: 2829–2849. https://doi.org/10.1039/d0fo03213a.
9. Huang Y, Zhao M, Chen X, et al. (2023) Tryptophan Metabolism in Central Nervous System Diseases: Pathophysiology and Potential Therapeutic Strategies. Aging Dis. 14: 858–878. https://doi.org/10.14336/AD.2022.0916.
10. Pandi-Perumal SR, Trakht I, Srinivasan V, et al. (2008) Physiological effects of melatonin: role of melatonin receptors and signal transduction pathways. Prog. Neurobiol. 85: 335–353. https://doi.org/10.1016/j.pneurobio.2008.04.001.
11. Grao-Cruces E, Calvo JR, Maldonado-Aibar MD, et al. (2023) Mediterranean Diet and Melatonin: A Systematic Review. Antioxidants (Basel, Switzerland) 12: 264. https://doi.org/10.3390/antiox12020264.
12. Cecon E, Oishi A, Jockers R (2018) Melatonin receptors: molecular pharmacology and signalling in the context of system bias. Br. J. Pharmacol. 175: 3263–3280. https://doi.org/10.1111/bph.13950.
13. Yu L-M, Di W-C, Dong X, et al. (2018) Melatonin protects diabetic heart against ischemia-reperfusion injury, role of membrane receptor-dependent cGMP-PKG activation. Biochim. Biophys. acta Mol. basis Dis. 1864: 563–578. https://doi.org/10.1016/j.bbadis.2017.11.023.
14. Del Castillo-Vaquero A, Salido GM, Gonzalez A (2010) Melatonin induces calcium release from CCK-8- and thapsigargin-sensitive cytosolic stores in pancreatic AR42J cells. J. Pineal Res. 49: 256–263. https://doi.org/10.1111/j.1600-079X.2010.00790.x.
15. Bach AG, Wolgast S, Mühlbauer E, Peschke E (2005) Melatonin stimulates inositol-1,4,5-trisphosphate and Ca2+ release from INS1 insulinoma cells. J. Pineal Res. 39: 316–323. https://doi.org/10.1111/j.1600-079X.2005.00253.x.
16. Argueta J, Solís-Chagoyán H, Estrada-Reyes R, et al. (2022) Further evidence of the melatonin calmodulin interaction: Effect on CaMKII activity. Int. J. Mol. Sci. 23: 2479. https://doi.org/10.3390/ijms23052479.
17. Ma H, Kang J, Fan W, et al. (2021) ROR: Nuclear receptor for melatonin or not? Molecules 26: 2693. https://doi.org/10.3390/molecules26092693.
18. Liu L, Labani N, Cecon E, Jockers R (2019) Melatonin target proteins: too many or not enough? Front. Endocrinol. (Lausanne) 10: 791. https://doi.org/10.3389/fendo.2019.00791.
19. Hacışevki A, Baba B (2018) An overview of melatonin as an antioxidant molecule: A biochemical approach. In: Drăgoi CM, Nicolae AC (eds) Melatonin. IntechOpen, Rijeka.
20. Hardeland R (2017) Melatonin and the electron transport chain. Cell Mol. Life Sci. 74: 3883–3896. https://doi.org/10.1007/s00018-017-2615-9.
21. Zhang H-M, Zhang Y (2014) Melatonin: a well-documented antioxidant with conditional pro-oxidant actions. J. Pineal Res. 57: 131–146. https://doi.org/10.1111/jpi.12162.
22. Mühlbauer E, Gross E, Labucay K, et al. (2009) Loss of melatonin signalling and its impact on circadian rhythms in mouse organs regulating blood glucose. Eur. J. Pharmacol. 606: 61–71. https://doi.org/10.1016/j.ejphar.2009.01.029.
23. Gonzalez A, Del Castillo-Vaquero A, Miro-Moran A, et al. (2011) Melatonin reduces pancreatic tumor cell viability by altering mitochondrial physiology. J. Pineal Res. 50: 250–260. https://doi.org/10.1111/j.1600-079X.2010.00834.x.
24. Jones DP (2008) Radical-free biology of oxidative stress. Am. J. Physiol. Cell. Physiol. 295: C849-68. https://doi.org/10.1152/ajpcell.00283.2008.
25. Graciano MFR, Valle MMR, Kowluru A, et al. (2011) Regulation of insulin secretion and reactive oxygen species production by free fatty acids in pancreatic islets. Islets 3: 213–223. https://doi.org/10.4161/isl.3.5.15935.
26. Fu Z, Gilbert ER, Liu D (2013) Regulation of insulin synthesis and secretion and pancreatic Beta-cell dysfunction in diabetes. Curr. Diabetes Rev. 9: 25–53.
27. Su S, Zhao Q, Dan L, et al. (2022) Inhibition of miR-146a-5p and miR-8114 in insulin-secreting cells contributes to the protection of melatonin against stearic acid-induced cellular senescence by targeting Mafa. Endocrinol. Metab. (Seoul, Korea) 37: 901–917. https://doi.org/10.3803/EnM.2022.1565.
28. Fabiś M, Pruszyńska E, Maćkowiak P (2002) In vivo and in situ action of melatonin on insulin secretion and some metabolic implications in the rat. Pancreas 25: 166–169. https://doi.org/10.1097/00006676-200208000-00009.
29. Tapia JA, Salido GM, González A (2010) Ethanol consumption as inductor of pancreatitis. World J. Gastrointest. Pharmacol. Ther. 1: 3–8. https://doi.org/10.4292/wjgpt.v1.i1.3.
30. Pandol SJ, Gorelick FS, Gerloff A, Lugea A (2010) Alcohol abuse, endoplasmic reticulum stress and pancreatitis. Dig. Dis. 28: 776–782. https://doi.org/10.1159/000327212.
31. Kurhaluk N (2021) Alcohol and melatonin. Chronobiol. Int. 38: 785–800. https://doi.org/10.1080/07420528.2021.1899198.
32. González A, Núñez AM, Granados MP, et al. (2006) Ethanol impairs CCK-8-evoked amylase secretion through Ca2+-mediated ROS generation in mouse pancreatic acinar cells. Alcohol 38: 51–57. https://doi.org/10.1016/j.alcohol.2006.03.002.
33. Bhardwaj P, Yadav RK (2013) Chronic pancreatitis: role of oxidative stress and antioxidants. Free Radic. Res. 47: 941–949. https://doi.org/10.3109/10715762.2013.804624.
34. Belyaev O, Herzog T, Munding J, et al. (2011) Protective role of endogenous melatonin in the early course of human acute pancreatitis. J. Pineal Res. 50: 71–77. https://doi.org/10.1111/j.1600-079X.2010.00811.x.
35. Hall JC, Crawford HC (2014) The conspiracy of autophagy, stress and inflammation in acute pancreatitis. Curr. Opin. Gastroenterol. 30: 495–499. https://doi.org/10.1097/MOG.0000000000000097.
36. Tang H, Zhang X, Huang J, et al. (2024) Phthalate and gallstones: the mediation of insulin. Front. public Heal. 12: 1401420. https://doi.org/10.3389/fpubh.2024.1401420.
37. Binker MG, Cosen-Binker LI (2014) Acute pancreatitis: the stress factor. World J. Gastroenterol. 20: 5801–5807. https://doi.org/10.3748/wjg.v20.i19.5801.
38. Santofimia-Castaño P, Ruy DC, Salido GM, González A (2013) Melatonin modulates Ca2+ mobilization and amylase release in response to cholecystokinin octapeptide in mouse pancreatic acinar cells. J. Physiol. Biochem. 69: 897–908. https://doi.org/10.1007/s13105-013-0267-2.
39. Maddatu J, Anderson-Baucum E, Evans-Molina C (2017) Smoking and the risk of type 2 diabetes. Transl. Res. 184: 101–107. https://doi.org/10.1016/j.trsl.2017.02.004.
40. Li T, Ni L, Zhao Z, et al. (2018) Melatonin attenuates smoking-induced hyperglycemia via preserving insulin secretion and hepatic glycogen synthesis in rats. J. Pineal Res. 64: e12475. https://doi.org/10.1111/jpi.12475.
41. Pandol SJ, Gottlieb RA (2022) Calcium, mitochondria and the initiation of acute pancreatitis. Pancreatol. Off. J. Int. Assoc. Pancreatol. 22: 838–845. https://doi.org/10.1016/j.pan.2022.07.011.
42. Shi C, Andersson R, Zhao X, Wang X (2005) Potential role of reactive oxygen species in pancreatitis-associated multiple organ dysfunction. Pancreatol. Off. J. Int. Assoc. Pancreatol. 5: 492–500. https://doi.org/10.1159/000087063.
43. Criddle DN (2016) Reactive oxygen species, Ca(2+) stores and acute pancreatitis; a step closer to therapy? Cell Calcium 60: 180–189. https://doi.org/10.1016/j.ceca.2016.04.007.
44. Pallagi P, Madácsy T, Varga Á, Maléth J (2020) Intracellular Ca(2+) Signalling in the Pathogenesis of Acute Pancreatitis: Recent Advances and Translational Perspectives. Int. J. Mol. Sci. 21. https://doi.org/10.3390/ijms21114005.
45. Jaworek J, Konturek SJ (2014) Hormonal protection in acute pancreatitis by ghrelin, leptin and melatonin. World J. Gastroenterol. 20: 16902–16912. https://doi.org/10.3748/wjg.v20.i45.16902.
46. Santofimia-Castaño P, Ruy DC, Fernandez-Bermejo M, et al. (2014) Pharmacological dose of melatonin reduces cytosolic calcium load in response to cholecystokinin in mouse pancreatic acinar cells. Mol. Cell. Biochem. 397: 75–86. https://doi.org/10.1007/s11010-014-2174-4.
47. Santofimia-Castaño P, Ruy DC, Salido GM, González A (2013) Melatonin modulates Ca2+ mobilization and amylase release in response to cholecystokinin octapeptide in mouse pancreatic acinar cells. J. Physiol. Biochem. 69: 897–908. https://doi.org/10.1007/s13105-013-0267-2.
48. Ma Q (2013) Role of nrf2 in oxidative stress and toxicity. Annu. Rev. Pharmacol. Toxicol. 53: 401–426. https://doi.org/10.1146/annurev-pharmtox-011112-140320.
49. Niture SK, Kaspar JW, Shen J, Jaiswal AK (2010) Nrf2 signaling and cell survival. Toxicol. Appl. Pharmacol. 244: 37–42. https://doi.org/10.1016/j.taap.2009.06.009.
50. Santofimia-Castaño P, Clea Ruy D, Garcia-Sanchez L, et al. (2015) Melatonin induces the expression of Nrf2-regulated antioxidant enzymes via PKC and Ca2+ influx activation in mouse pancreatic acinar cells. Free Radic. Biol. Med. 87: 226–236. https://doi.org/10.1016/j.freeradbiomed.2015.06.033.
51. Atlanta: American Cancer Society I Cancer Facts & Figures 2023.
52. Kleeff J, Korc M, Apte M, et al. (2016) Pancreatic cancer. Nat. Rev. Dis. Prim. 2: 16022. https://doi.org/10.1038/nrdp.2016.22.
53. Orth M, Metzger P, Gerum S, et al. (2019) Pancreatic ductal adenocarcinoma: biological hallmarks, current status, and future perspectives of combined modality treatment approaches. Radiat. Oncol. 14: 141. https://doi.org/10.1186/s13014-019-1345-6.
54. Friend C, Parajuli P, Razzaque MS, Atfi A (2023) Deciphering epithelial-to-mesenchymal transition in pancreatic cancer. Adv. Cancer Res. 159: 37–73. https://doi.org/10.1016/bs.acr.2023.02.008.
55. Krüger A (2015) Premetastatic niche formation in the liver: emerging mechanisms and mouse models. J. Mol. Med. (Berl) 93: 1193–1201. https://doi.org/10.1007/s00109-015-1342-7.
56. Benkhaled S, Peters C, Jullian N, et al. (2023) Combination, modulation and interplay of modern radiotherapy with the tumor microenvironment and targeted therapies in pancreatic cancer: Which candidates to boost radiotherapy? Cancers (Basel) 15: 768. https://doi.org/10.3390/cancers15030768.
57. Bhoopathi P, Mannangatti P, Das SK, et al. (2023) Chemoresistance in pancreatic ductal adenocarcinoma: Overcoming resistance to therapy. Adv. Cancer Res. 159: 285–341. https://doi.org/10.1016/bs.acr.2023.02.010.
58. Hagel KR, Arafeh R, Gang S, et al. (2023) Systematic interrogation of tumor cell resistance to chimeric antigen receptor T-cell therapy in pancreatic cancer. Cancer Res. 83: 613–625. https://doi.org/10.1158/0008-5472.CAN-22-2245.
59. Gillen S, Schuster T, Meyer Zum Büschenfelde C, et al. (2010) Preoperative/neoadjuvant therapy in pancreatic cancer: a systematic review and meta-analysis of response and resection percentages. PLoS Med. 7: e1000267. https://doi.org/10.1371/journal.pmed.1000267.
60. Teague A, Lim K-H, Wang-Gillam A (2015) Advanced pancreatic adenocarcinoma: a review of current treatment strategies and developing therapies. Ther. Adv. Med. Oncol. 7: 68–84. https://doi.org/10.1177/1758834014564775.
61. Li W, Wu J, Li Z, et al. (2016) Melatonin induces cell apoptosis in Mia PaCa-2 cells via the suppression of nuclear factor-κB and activation of ERK and JNK: A novel therapeutic implication for pancreatic cancer. Oncol. Rep. 36: 2861–2867. https://doi.org/10.3892/or.2016.5100.
62. Ju H-Q, Li H, Tian T, et al. (2016) Melatonin overcomes gemcitabine resistance in pancreatic ductal adenocarcinoma by abrogating nuclear factor-κB activation. J. Pineal Res. 60: 27–38. https://doi.org/10.1111/jpi.12285.
63. Leja-Szpak A, Jaworek J, Szklarczyk J, et al. (2007) Melatonin stimulates HSP27 phosphorylation in human pancreatic carcinoma cells (PANC-1). J. Physiol. Pharmacol. an Off J Polish Physiol Soc 58: Suppl 3:177–188.
64. Leja-Szpak A, Nawrot-Porąbka K, Góralska M, et al. (2018) Melatonin and its metabolite N1-acetyl-N2-formyl-5-methoxykynuramine (afmk) enhance chemosensitivity to gemcitabine in pancreatic carcinoma cells (PANC-1). Pharmacol. Rep. 70: 1079–1088. https://doi.org/10.1016/j.pharep.2018.05.007.
65. Cui P, Yu M, Peng X, et al. (2012) Melatonin prevents human pancreatic carcinoma cell PANC-1-induced human umbilical vein endothelial cell proliferation and migration by inhibiting vascular endothelial growth factor expression. J. Pineal Res. 52: 236–243. https://doi.org/10.1111/j.1600-079X.2011.00933.x.
66. Xu C, Wu A, Zhu H, et al. (2013) Melatonin is involved in the apoptosis and necrosis of pancreatic cancer cell line SW-1990 via modulating of Bcl-2/Bax balance. Biomed. Pharmacother. 67: 133–139. https://doi.org/10.1016/j.biopha.2012.10.005.
67. Uguz AC, Cig B, Espino J, et al. (2012) Melatonin potentiates chemotherapy-induced cytotoxicity and apoptosis in rat pancreatic tumor cells. J. Pineal Res. 53: 91–98. https://doi.org/10.1111/j.1600-079X.2012.00974.x.
68. Perfilyeva Y V, Ostapchuk YO, Abdolla N, et al. (2019) Exogenous melatonin up-regulates expression of cd62l by lymphocytes in aged mice under inflammatory and non-inflammatory conditions. Immunol. Invest. 48: 632–643. https://doi.org/10.1080/08820139.2019.1586918.
69. Piersma B, Hayward MK, Weaver VM (2020) Fibrosis and cancer: A strained relationship. Biochim. Biophys. acta Rev. cancer. 1873: 188356. https://doi.org/10.1016/j.bbcan.2020.188356
70. Fang Z, Jung KH, Yan HH, et al. (2018) Melatonin synergizes with sorafenib to suppress pancreatic cancer via melatonin receptor and PDGFR-β/STAT3 pathway. Cell. Physiol. Biochem. 47: 1751–1768. https://doi.org/10.1159/000491058.
71. Padillo FJ, Ruiz-Rabelo JF, Cruz A, et al. (2010) Melatonin and celecoxib improve the outcomes in hamsters with experimental pancreatic cancer. J. Pineal Res. 49: 264–270. https://doi.org/10.1111/j.1600-079X.2010.00791.x.
72. Chan Y-T, Tan H-Y, Lu Y, et al. (2023) Pancreatic melatonin enhances anti-tumor immunity in pancreatic adenocarcinoma through regulating tumor-associated neutrophils infiltration and NETosis. Acta Pharm. Sin. B. 13: 1554–1567. https://doi.org/10.1016/j.apsb.2023.01.020.
73. Sánchez-Barceló AGGNREJ (2019) Clinical uses of melatonin: evaluation of human trials on cancer treatment. Melatonin Res. 2: 47–69. https://doi.org/https://doi.org/10.32794/mr11250021.
74. Ferdek PE, Krzysztofik D, Stopa KB, et al. (2022) When healing turns into killing - the pathophysiology of pancreatic and hepatic fibrosis. J. Physiol. 600: 2579–2612. https://doi.org/10.1113/JP281135.
75. Kleeff J, Whitcomb DC, Shimosegawa T, et al. (2017) Chronic pancreatitis. Nat. Rev. Dis. Prim. 3: 17060. https://doi.org/10.1038/nrdp.2017.60.
76. Hosein AN, Brekken RA, Maitra A (2020) Pancreatic cancer stroma: an update on therapeutic targeting strategies. Nat. Rev. Gastroenterol. Hepatol. 17: 487–505. https://doi.org/10.1038/s41575-020-0300-1.
77. Sherman MH (2018) Stellate Cells in Tissue Repair, Inflammation, and Cancer. Annu. Rev. Cell. Dev. Biol. 34: 333–355. https://doi.org/10.1146/annurev-cellbio-100617-062855.
78. Apte M, Pirola RC, Wilson JS (2015) Pancreatic stellate cell: physiologic role, role in fibrosis and cancer. Curr. Opin. Gastroenterol. 31:_416–423. https://doi.org/10.1097/MOG.0000000000000196.
79. Santofimia-Castaño P, Garcia-Sanchez L, Ruy DC, et al. (2015) Melatonin induces calcium mobilization and influences cell proliferation independently of MT1/MT2 receptor activation in rat pancreatic stellate cells. Cell Biol. Toxicol. 31: 95–110. https://doi.org/10.1007/s10565-015-9297-6.
80. Estaras M, Moreno N, Santofimia-Castaño P, et al. (2019) Melatonin induces reactive oxygen species generation and changes in glutathione levels and reduces viability in human pancreatic stellate cells. J. Physiol. Biochem. 75:_185–197. https://doi.org/10.1007/s13105-019-00671-x.
81. Estaras M, Peña FJ, Tapia JA, et al. (2020) Melatonin modulates proliferation of pancreatic stellate cells through caspase-3 activation and changes in cyclin A and D expression. J. Physiol. Biochem. 76: 345–355. https://doi.org/10.1007/s13105-020-00740-6.
82. Estaras M, Martinez-Morcillo S, García A, et al. (2020) Pancreatic stellate cells exhibit adaptation to oxidative stress evoked by hypoxia. Biol. cell. 112: 280–299. https://doi.org/10.1111/boc.202000020.
83. Estaras M, Ortiz-Placin C, Castillejo-Rufo A, et al. (2023) Melatonin controls cell proliferation and modulates mitochondrial physiology in pancreatic stellate cells. J. Physiol. Biochem. 79: 235–249. https://doi.org/10.1007/s13105-022-00930-4.
84. Que J, Lian Q, El Oakley RM, et al. (2007) PI3 K/Akt/mTOR-mediated translational control regulates proliferation and differentiation of lineage-restricted RoSH stem cell lines. J. Mol. Signal. 2: 9. https://doi.org/10.1186/1750-2187-2-9.
85. Estaras M, Martinez R, Garcia A, et al. (2022) Melatonin modulates metabolic adaptation of pancreatic stellate cells subjected to hypoxia. Biochem. Pharmacol. 202: 115118. https://doi.org/10.1016/j.bcp.2022.115118.
86. Gonzalez A, Estaras M, Martinez-Morcillo S, et al. (2020) Melatonin modulates red-ox state and decreases viability of rat pancreatic stellate cells. Sci. Rep. 10: 6352. https://doi.org/10.1038/s41598-020-63433-6.
87. Jing X, Yang F, Shao C, et al. (2019) Role of hypoxia in cancer therapy by regulating the tumor microenvironment. Mol. Cancer. 18: 157. https://doi.org/10.1186/s12943-019-1089-9.
88. Infantino V, Santarsiero A, Convertini P, et al. (2021) Cancer cell metabolism in hypoxia: role of HIF-1 as key regulator and therapeutic target. Int. J. Mol. Sci. 22: https://doi.org/10.3390/ijms22115703.
89. Estaras M, Gonzalez A (2021) Modulation of cell physiology under hypoxia in pancreatic cancer. World J. Gastroenterol. 27: 4582–4602. https://doi.org/10.3748/wjg.v27.i28.4582.
90. Cheng J, Yang H-L, Gu C-J, et al. (2019) Melatonin restricts the viability and angiogenesis of vascular endothelial cells by suppressing HIF-1α/ROS/VEGF. Int. J. Mol. Med. 43: 945–955. https://doi.org/10.3892/ijmm.2018.4021.
91. Estaras M, Gonzalez-Portillo MR, Martinez R, et al. (2021) Melatonin modulates the antioxidant defenses and the expression of proinflammatory mediators in pancreatic stellate cells subjected to hypoxia. Antioxidants (Basel, Switzerland) 10: 577. https://doi.org/10.3390/antiox10040577.
92. Estaras M., Gonzalez-Portillo MR., Fernandez-Bermejo, M.; Mateos JM., et al. (2021) Melatonin induces apoptosis and modulates cyclin expression and mapk phosphorylation in pancreatic stellate cells subjected to hypoxia. Int. J. Mol. Sci. 22: 5555. https://doi.org/10.3390/ijms22115555.
93. San-Miguel B, Crespo I, Sánchez DI, et al. (2015) Melatonin inhibits autophagy and endoplasmic reticulum stress in mice with carbon tetrachloride-induced fibrosis. J. Pineal Res. 59: 151–162. https://doi.org/10.1111/jpi.12247.
94. Tanoğlu EG, Tanoğlu A, Meriçöz Aydın MÇ, Esen MF (2021) Melatonin has favorable preventive effects on experimental chronic pancreatitis rat model. Turkish J. Med. Sci. 51: 2734–2740. https://doi.org/10.3906/sag-2103-134.
95. Jiang W, Jin L, Ju D, et al. (2022) The pancreatic clock is a key determinant of pancreatic fibrosis progression and exocrine dysfunction. Sci. Transl. Med. 14: eabn3586. https://doi.org/10.1126/scitranslmed.abn3586.
96. Primavera M, Giannini C, Chiarelli F (2020) Prediction and prevention of type 1 diabetes. Front. Endocrinol. (Lausanne) 11: 248. https://doi.org/10.3389/fendo.2020.00248.
97. Chatterjee S, Khunti K, Davies MJ (2017) Type 2 diabetes. Lancet (London, England) 389: 2239–2251. https://doi.org/10.1016/S0140-6736(17)30058-2.
98. Costes S, Boss M, Thomas AP, Matveyenko A V (2015) Activation of melatonin signaling promotes β-cell survival and function. Mol. Endocrinol. 29: 682–692. https://doi.org/10.1210/me.2014-1293.
99. Park J-H, Shim H-M, Na A-Y, et al. (2014) Melatonin prevents pancreatic β-cell loss due to glucotoxicity: The relationship between oxidative stress and endoplasmic reticulum stress. J. Pineal Res. 56: 143–153. https://doi.org/10.1111/jpi.12106.
100. Ramracheya RD, Muller DS, Squires PE, et al. (2008) Function and expression of melatonin receptors on human pancreatic islets. J. Pineal Res. 44: 273–279. https://doi.org/10.1111/j.1600-079X.2007.00523.x.
101. Tao S, Yang Y, Fan Y, et al. (2022) Melatonin protects against nonylphenol caused pancreatic β-cells damage through MDM2-P53-P21 axis. Toxicol. Res. (Camb) 11: 391–401. https://doi.org/10.1093/toxres/tfac016.
102. Lee YH, Jung HS, Kwon MJ, et al. (2020) Melatonin protects INS-1 pancreatic β-cells from apoptosis and senescence induced by glucotoxicity and glucolipotoxicity. Islets 12: 87–98. https://doi.org/10.1080/19382014.2020.1783162.
103. Farid A, Moussa P, Youssef M, et al. (2022) Melatonin relieves diabetic complications and regenerates pancreatic beta cells by the reduction in NF-kB expression in streptozotocin induced diabetic rats. Saudi J. Biol. Sci. 29: 103313. https://doi.org/10.1016/j.sjbs.2022.103313.
104. Abdulwahab DA, El-Missiry MA, Shabana S, et al. (2021) Melatonin protects the heart and pancreas by improving glucose homeostasis, oxidative stress, inflammation and apoptosis in T2DM-induced rats. Heliyon 7: e06474. https://doi.org/10.1016/j.heliyon.2021.e06474.
105. El Aasar H, Rashed L, El Sadik A, et al. (2022) The role of the adipose tissue-derived mesenchymal stem cells enriched with melatonin in pancreatic cellular regeneration. Folia Morphol. (Warsz) 81: 931–941. https://doi.org/10.5603/FM.a2021.0093.
106. Gomes PRL, Vilas-Boas EA, Leite E de A, et al. (2021) Melatonin regulates maternal pancreatic remodeling and B-cell function during pregnancy and lactation. J. Pineal Res. 71: e12717. https://doi.org/10.1111/jpi.12717.
107. Preethy S, Yamamoto N, Liem N, et al. (2023) Role of gut microbiome homeostasis, integrity of the intestinal epithelial cells, and the (endogenous) butyrate in enduring a healthy long liFE. Georgian Med. News. 336: 73–78.
108. Pedersen SS, Ingerslev LR, Olsen M, et al. (2024) Butyrate functions as a histone deacetylase inhibitor to protect pancreatic beta cells from IL-1β-induced dysfunction. FEBS J. 291: 566–583. https://doi.org/10.1111/febs.17005.
109. Jin CJ, Engstler AJ, Sellmann C, et al. (2016) Sodium butyrate protects mice from the development of the early signs of non-alcoholic fatty liver disease: role of melatonin and lipid peroxidation. Br. J. Nutr. 116: 1682–1693. https://doi.org/10.1017/S0007114516004025.
110. Anderson G, Maes M (2020) Gut dysbiosis dysregulates central and systemic homeostasis via suboptimal mitochondrial function: assessment, treatment and classification implications. Curr. Top. Med. Chem. 20: 524–539. https://doi.org/10.2174/1568026620666200131094445.
111. Kanika G, Khan S, Jena G (2015) Sodium butyrate ameliorates l-arginine-induced pancreatitis and associated fibrosis in wistar rat: Role of inflammation and nitrosative stress. J. Biochem. Mol. Toxicol. 29: 349–359. https://doi.org/10.1002/jbt.21698.
112. Sanaei M, Kavoosi F (2022) Effect of sodium butyrate on p16INK4a, p14ARF, p15INK4b, class I HDACs (HDACs 1, 2, 3) class II HDACs (HDACs 4, 5, 6), cell growth inhibition and apoptosis induction in pancreatic cancer AsPC-1 and colon cancer HCT-116 cell lines. Asian Pac. J. Cancer Prev. 23: 795–802. https://doi.org/10.31557/APJCP.2022.23.3.795.
113. Anderson G (2023) Gut Microbiome and circadian interactions with platelets across human diseases, including Alzheimer’s disease, amyotrophic lateral sclerosis, and cancer. Curr. Top. Med. Chem. 23:_2699–2719. https://doi.org/10.2174/0115680266253465230920114223.
114. Wang X, Zhan W, Huang L, et al. (2023) The effect of anxiety and depression on the health-related quality of life of severe acute pancreatitis survivors: structural equation modeling approach. Front. psychiatry 14: 1160807. https://doi.org/10.3389/fpsyt.2023.1160807.
115. Hirth M, Göltl P, Weiss C, et al. (2021) Association between pancreatic burnout and liver cirrhosis in alcoholic chronic pancreatitis. Digestion 102: 887–894. https://doi.org/10.1159/000516482.
116. Liu L, Han S, Xiao X, et al. (2022) Glucocorticoid-induced microRNA-378 signaling mediates the progression of pancreatic cancer by enhancing autophagy. Cell. Death. Dis. 13: 1052. https://doi.org/10.1038/s41419-022-05503-3.
117. Kupczyk D, Bilski R, Kozakiewicz M, et al. (2022) 11β-HSD as a new target in pharmacotherapy of metabolic diseases. Int. J. Mol. Sci. 23. https://doi.org/10.3390/ijms23168984.
118. Quiros I, Mayo JC, Garcia-Suarez O, et al. (2008) Melatonin prevents glucocorticoid inhibition of cell proliferation and toxicity in hippocampal cells by reducing glucocorticoid receptor nuclear translocation. J. Steroid Biochem. Mol. Biol. 110: 116–124. https://doi.org/10.1016/j.jsbmb.2008.02.009.
119. Anderson G (2024) Polycystic ovary syndrome pathophysiology: Integrating systemic, CNS and circadian processes. Front. Biosci. (Landmark Ed) 29: 24. https://doi.org/10.31083/j.fbl2901024.
120. Anderson G (2019) Gut dysbiosis dysregulates central and systemic homeostasis via decreased melatonin and suboptimal mitochondria functioning: pathoetiological and pathophysiological implications. Melatonin Res. 2: 70–85. https://doi.org/10.32794/mr11250022.
121. Anderson G (2023) Why are aging and stress associated with dementia, cancer, and other diverse medical conditions? Role of pineal melatonin interactions with gut microbiome butyrate in HPA axis and cortisol awakening response regulation: Possible role of BAG-1. Melatonin Res. 6: 345–371. https://doi.org/10.32794/mr112500158.
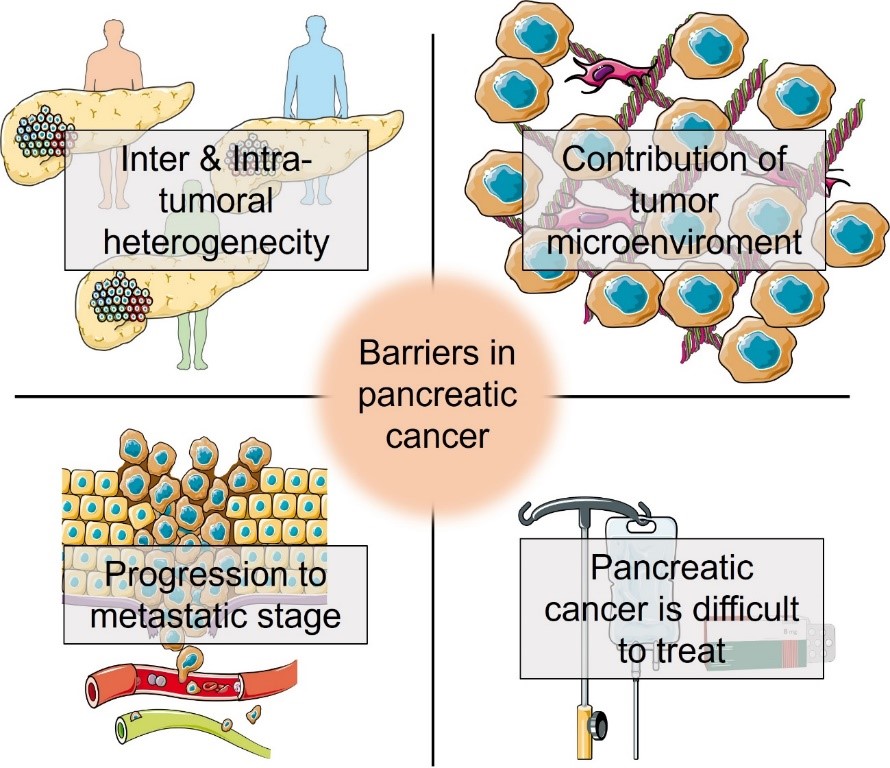

This work is licensed under a Creative Commons Attribution 4.0 International License.
For all articles published in Melatonin Res., copyright is retained by the authors. Articles are licensed under an open access Creative Commons CC BY 4.0 license, meaning that anyone may download and read the paper for free. In addition, the article may be reused and quoted provided that the original published version is cited. These conditions allow for maximum use and exposure of the work, while ensuring that the authors receive proper credit.
In exceptional circumstances articles may be licensed differently. If you have specific condition (such as one linked to funding) that does not allow this license, please mention this to the editorial office of the journal at submission. Exceptions will be granted at the discretion of the publisher.