Plasticity of glucose metabolism in activated immune cells: advantages for melatonin inhibition of COVID-19 disease
Melatonin mechanisms in COVID-19
Abstract
COVID-19 has infected hundreds of thousands and killed tens of thousands of people worldwide and it continues to ravage societies as well as fiscal and economic stability of several countries. Currently, several drugs that were designed for other conditions have been repurposed to counter the COVID-19 pandemic. Some have modest efficacy in resisting this disease, but all of them have significant toxicity. Several pharmaceutical companies are rushing to develop vaccines, but their availability is 8 to 12 months in the future. In the meantime, readily available and affordable molecules that will have utility as COVID-19 antidotes are being sought. Recently, several groups independently and almost simultaneously proposed that melatonin should be considered for this purpose and several trials are underway to test whether melatonin is a reliable candidate drug for COVID-19 treatment. In this brief review, we described some potential mechanisms by which melatonin may work to protect against a COVID-19 infection. Of particular note is the likely ability of melatonin to force activated immune cells to abandon aerobic glycolysis in favor of mitochondrial oxidative phosphorylation. Aerobic glycolysis gives proinflammatory activated immune cells, for example, macrophages, an opportunity to produce increased amounts of cytokines which are released as the cytokine storm. The associated significant increase in oxidative stress is accepted as a major contributing factor to the bronchoalveolar dysfunction and pneumonia that occurs in COVID-19-infected individuals. Melatonin, via similar processes, may reduce the formation of proinflammatory M1 macrophages and convert them to macrophages of the M2 phenotype, which are anti-inflammatory. These are not the only mechanisms by which melatonin may protect against the deadly COVID-19 pandemic. Melatonin could also be given as an adjuvant with other toxic pharmaceutical agents with a high likelihood it would reduce their side effects.
References
2. Thompson BT, Chambers RC, Liu KD. (2017) Acute respiratory distress syndrome.
N. Engl. J. Med. 377: 1904-1905.
3. Tisoncik JR, Korth MJ, Simmons CP, et al. (2012) Into the eye of the cytokine storm. Microbiol. Mol. Biol. Rev. 76: 16-32.
4. Cheung CY, Poon LLM, Ng IHY, et al. (2005) Cytokine responses in severe acute respiratory syndrome coronavirus-infected macrophages in vitro: possible relevance to pathogenesis. J. Virol. 79: 7819-7826.
5. Chu H, Zhou J, Wong BHY, et al. (2016) Middle East respiratory syndrome coronavirus efficiently infects human primary T lymphocytes and activates the extrinsic and intrinsic apoptotic pathways. J. Infect. Dis. 213: 904:914.
6. Cipolla-Neto J, Amaral F. (2018) Melatonin as a hormone: new physiological and clinical insights. Endocr. Rev. 39: 990-1028.
7. Tricoire H, Locatelli A, Chemineau P, et al. (2002) Melatonin enters the cerebrospinal fluid through the pineal recess. Endocrinology 143: 84-90.
8. Reiter RJ, Tan DX, Kim SJ, et al., (2014) Delivery of pineal melatonin to the brain and SCN: role of canaliculi, cerebrospinal fluid, tanycytes and Virchow-Robin perivascular spaces. Brain Struct. Funct. 219: 1873-1887.
9. Acuna-Castroviejo D, Escames G, Venegas C, et al. (2014) Extrapineal melatonin: sources, regulation, and potential functions. Cell. Mol. Life Sci. 71: 2997-3025.
10. Poeggeler B, Hardeland R. (1994) Detection and quantification of melatonin in a dinoflagellate, Gonyaulax polyedra: solutions to the problems of methoxyindole destruction in non-vertebrate material. J. Pineal Res. 17: 1-10.
11. Manchester LC, Poeggeler B, Alvares FL, et al. (1995) Melatonin immunoreactivity in the photosynthetic prokaryote Rhodospirillum rubrum: implications for an ancient antioxidant system. Cell. Mol. Biol. Res. 41: 391-395.
12. Zimorski V, Ku C, Martin WF, et al. (2014) Endosymbiotic theory for organelle origins. Curr. Opin. Microbiol. 22: 38-48.
13. Tan DX, Manchester LC, Liu X, et al. (2013) Mitochondria and chloroplasts as the original sites of melatonin synthesis: a hypothesis related to melatonin’s primary function in evolution in eukaryotes. J. Pineal Res. 54: 127-138.
14. Venegas C, Garcia JA, Escames G, et al. (2012) Extrapineal melatonin: analysis of its subcellular distribution and daily fluctuations. J. Pineal Res. 52: 217-227.
15. He C, Wang J, Zhang Z, et al. (2016) Mitochondria synthesize melatonin to ameliorate its function and improve mice oocyte’s quality under in vitro conditions. Int. J. Mol. Sci. 17: E939.
16. Suofu Y, Jean Alphonse FG, Jia J, et al. (2017) Dual role of mitochondria in producing melatonin and driving GPCR signaling to block cytochrome c release. Proc. Nat. Acad. Sci. USA 114: E7997-E8006.
17. Reiter RJ, Sharma R, Ma Q, et al. (2020) Circadian and non-circadian melatonin: influence on glucose metabolism in cancer cells. J. Curr. Sci. Technol. 10: 85-98.
18. Reiter RJ, Ma Q, Sharma R. (2020) Melatonin in mitochondria: mitigating clear and present dangers. Physiology (Bethesda) 35: 86-95.
19. Reiter RJ, Sharma R, Ma Q, et al. (2020) Melatonin inhibits Warburg-dependent cancer by redirecting glucose oxidation to the mitochondria: a mechanistic hypothesis. Cell. Mol. Life Sci., in press.
20. Yamamoto T, Takano N, Ishiwata K, et al. (2018) Reduced methylation of PFKFB3 in cancer cells shunts glucose towards the pentose phosphate pathway. Nat. Commun. 5: 3480.
21. Okatani Y, Wakatsuki A, Reiter RJ, et al. (2003) Acutely administered melatonin restores hepatic mitochondrial physiology in old mice. Int. J. Biochem. Cell. Biol. 35: 367-375.
22. Jou MJ, Peng TI, Yu PZ, et al. (2007) Melatonin protects against common deletion of mitochondrial DNA-augmented mitochondria oxidative stress and apoptosis. J. Pineal Res. 43: 389-403.
23. Hevia D, Gonzalez-Menendez P, Quiros-Gonzalez I, et al. (2015) Melatonin uptake through glucose transporters: a new target for melatonin inhibition of cancer. J. Pineal Res. 58: 234-250.
24. Huo X, Wang C, Yu Z, et al. (2017) Human transporters, PEPT1/2, facilitate melatonin transportation into mitochondria of cancer cells: an implication of the therapeutic potential. J. Pineal Res. 62: 12390.
25. Acuna-Castroviejo D, Noguiera-Navarro MT, Reiter RJ, et al. (2018) Melatonin actions in the heart; more than a hormone. Melatonin Res. 1: 21-26.
26. Reiter RJ, Rosales-Corral S, Tan DX, et al. (2017) Melatonin as a mitochondria-targeted antioxidant: one of evolution’s best ideas. Cell. Mol. Life Sci. 74: 3863-3881.
27. Reiter RJ, Mayo JC, Tan DX, et al. (2016) Melatonin as an antioxidant: under promises but over delivers. J. Pineal Res. 61: 253-278.
28. Blask DE, Dauchy RT, Dauchy EM, et al. (2014) Light exposure at night disrupts host/cancer circadian regulatory dynamics: impact on the Warburg effect, lipid signaling and tumor growth prevention. PLoS One 9: e102776.
29. Tan DX, Reiter RJ. (2019) Mitochondria: the birth place, battle ground and the site of melatonin metabolism in cells. Melatonin Res. 2: 44-66.
30. Reiter RJ, Rosales-Corral S. (2019) Melatonin reprograms glucose metabolism in cancer cell mitochondria. Series Endocrinol. Diabetes Metab. 1: 52-61.
31. Reiter RJ, Sharma R, Ma Q, et al. (2019) Inhibition of pyruvate dehydrogenase kinase: a proposed mechanism by which melatonin causes cancer cells to overcome cytosolic glycolysis, reduce tumor biomass and reverse insensitivity to chemotherapy. Melatonin Res. 2: 105-119.
32. Courtnay R, Ngo DC, Malik N, et al. (2015) Cancer metabolism and the Warburg effect: the role of HIF-1 and PI3K. Mol. Biol. Rep. 42: 841-851.
33. Jardim-Perassi BV, Alexandre PA, Sonehara NM, et al. (2019) RNA-Seq transcriptome analysis shows anti-tumor actions of melatonin in a breast cancer xenograft model. Sci. Rep. 9: 966.
34. Ferreira LC, Orso F, Dettori D, et al. (2020) The role of melatonin on miRNAs modulation in triple-negative breast cancer cells. PLoS One 15: e0228062.
35. Zhao D, Yu Y, Shen Y, et al. (2019) Melatonin synthesis and function: evolutionary history in animals and plants. Front. Endocrinol. 10: 249.
36. Klein DC (2007) Arylalkylamine N-acetyltransferase: the timenzyme. J. Biol. Chem. 282: 4233-4237.
37. Anderson G. (2019) Daytime orexin and night-time melatonin regulation of mitochondria melatonin: roles in circadian oscillations systemically and centrally in breast cancer symptomatology. Melatonin Res. 2: 1-8.
38. Anderson G, Maes M, Markus RP, et al. (2015) Ebola virus: melatonin as a readily available treatment option. J. Med. Virol. 87: 537-543.
39. Anderson G, Reiter RJ. (2019) Glioblastoma: role of mitochondria N-acetylserotonin/melatonin ratio in mediating effects of miR-451 and aryl hydrocarbon receptor and in coordinating wider biochemical changes. Int. J. Tryptophan Res. 12: 1-9.
40. Arabi YM, Fowler R, Hayden FG. (2020) Critical care management of adults with community-acquired severe respiratory viral infection. Intensive Care Med. 46: 315-328.
41. Grant WB, Lahore H, McDonnell SL, et al. (2020) Evidence that vitamin D Supplementation could reduce risk of influenza and COVID-19 infection and deaths. Nutrients 12: E988.
42. Marik PE, Kory P, Varon J. (2020) Does vitamin D status impact mortality from SARS-CoV-2 infection? Med. Drug Discov. 29: 100041.
43. Martin-Gimenez VM, Inserra F, Tajer CE, et al. (2020) Lungs as target of COVID-19 infection: protective common molecular mechanisms of vitamin D and melatonin as a potential synergistic treatment. Life Sci. 254: 117808.
44. Wu C, Chen X, Cai Y, et al. (2020) Risk factors associated with acute respiratory distress syndrome and death in patients with coronavirus disease 2019 pneumonia in Wuhan, China. JAMA Intern. Med. 13: e200994.
45. Mehta P, McAuley DF, Brown M, et al. (2020) COVID-19: Consider cytokine storm syndromes and immunosuppression. Lancet 395: 1033-1934.
46. Martins E Jr, Ferreira AL, Skorupa AL, et al. (2004) Tryptophan consumption and indoleamines production in peritoneal cavity macrophages. J. Leukoc. Biol. 75: 1116-1121.
47. Muxel SM, Pires-Lapa MA, Monteiro AW, et al. (2012) NF-kB drives the synthesis of melatonin in RAW 264.7macrophages by inducing the transcription of the arylalkylamine N-acetyltransferase (AA-NAT) gene. PLoS One 7: E52010.
48. Muxel SM, Laranjeira-Silva MF, Carvalho-Sousa CE, et al. (2016) The RelA/cRel nuclear factor-kB (NF-kB) dimer, crucial for inflammation resolution, mediates the transcription of the key enzyme in melatonin synthesis in RAW 264:7 macrophages.
J. Pineal Res. 60: 394-404.
49. Carvalho-Sousa CE, Pereira EP, Kinker GS, et al. (2020) Immune-pineal axis protects rat lungs exposed to polluted air. J. Pineal Res. 68: e12636.
50. Gordon S. (1995) The macrophage. Bioessays 17: 977-986.
51. Osborn O, Olefsky JM (2012) The cellular and signaling networks linking the immune system and metabolism in disease. Nat. Med. 18: 363-374.
52. Gordon S, Martinez FO. (2010) Alternative activation of macrophages: mechanism and functions. Immunity 32: 593-604.
53. Rodriguez-Prados JC, Traves PG, Cuenca J, et al. (2010) Substrate fate in activated macrophages: a comparison between innate, classic, and alternative activation. J. Immunol. 185: 605-614.
54. Hardeland R. (2018) Melatonin and information-story of a double-edged blade. J. Pineal Res. 65: e12525.
55. Perez-Gonzalez A, Castaneda-Arriaga R, Alvarez-Idaboy JR, et al. (2019) Melatonin and its metabolites as chemical agents capable of directly repairing oxidized DNA. J. Pineal Res. 66: e12539.
56. Tan DX, Hardeland R. (2020) Potential utility of melatonin in deadly infectious diseases related to the overreaction of innate immune system response and destructive inflammation: focus on COVID-19. Melatonin Res. 3: 120-143.
57. Reiter RJ, Sharma R, Ma Q, et al. (2020) Melatonin inhibits COVID-19-induced cytokine storm by reversing aerobic glycolysis in immune cells: a mechanistic analysis. Med. Drug. Discov., in press.
58. Behrens EM, Koretzky GA (2017) Review: Cytokine storm syndrome: looking forward toward the precision medicine era. Arthritis Rheumatol. 69: 1135-1143.
59. Tugal D, Liao X, Jain MK. (2013) Transcriptional control of macrophage polarization. Arterioscler. Thromb. Vasc. Biol. 33: 1135-1144.
60. Essandoh K, Li Y, Huo J, et al. (2016) MiRNA-mediated macrophage polarization and its potential role in the regulation of inflammatory response. Shock 46: 122-131.
61. Tobias PS, Soldau K, Ulevitch RJ. (1989) Identification of a lipid A binding site in the acute phase reactant lipopolysaccharide binding protein. J. Biol. Chem. 264: 10867-10871.
62. Mauriz JL, Collado PS, Veneroso C, et al. (2013) A review of the molecular aspects of melatonin’s anti-inflammatory actions: recent insights and new perspectives. J. Pineal Res. 54: 1-14.
63. Liu Z, Gan L, Xu Y, et al. (2017) Melatonin alleviates inflammasome-induced pyroptosis through inhibiting NF-kB/GSDMD signal in mice adipose tissue. J. Pineal Res. 63: 12414.
64. Yi WJ, Kim TS. (2017) Melatonin protects mice against stress-induced inflammation through enhancement of M2 macrophage polarization. Int. Immunopharmacol. 48: 146-158.
65. Amici SA, Dong J, Guerau-de-Arellano M. (2017) Molecular mechanisms modulating the phenotype of macrophages and microglia. Front. Immunol. 8: 1520.
66. Carloni S, Favrais G, Saliba E, et al. (2016) Melatonin modulates neonatal brain inflammation through endoplasmic reticulum stress, autophagy and miR-34a/silent information regulator 1 pathway. J. Pineal Res. 61: 370-380.
67. Ding S, Lin N, Sheng X, et al. (2019) Melatonin stabilizes rupture-prone vulnerable plaques via regulating macrophage polarization in a nuclear circadian receptor RORα-dependent manner. J. Pineal Res. 67: e12581.
68. Xia Y, Chen S, Zeng S, et al. (2019) Melatonin in macrophage biology: current understanding and future perspectives. J. Pineal Res. 66: e12547.
69. Liu ZJ, Ran YY, Qie SY, et al. (2019) Melatonin protects against ischemic stroke by modulating microglia/macrophage polarization toward anti-inflammatory phenotype through STAT3 pathway. CNS Neurosci. Ther. 25: 1353-1362.
70. Thomas MC, Pickering RJ, Tsorotes D, et al. (2010) Genetic Ace2 deficiency accentuates vascular inflammation and atherosclerosis in the ApoE knockout mouse. Circ. Res. 107: 888-897.
71. McGonagle D, Sharif K, O’Regan A, et al. (2020) The role of cytokines including interleukin-6 in COVID-19 induced pneumonia and macrophage activation syndrome-like disease. Autoimmun. Rev. 2020: 102537.
72. He L, Ding Y, Zhang Q, et al. (2006) Expression of elevated levels of pro-inflammatory cytokines in SARS-CoV-infected ACE2+ cells in SARS patients: relation to acute lung injury and pathogenesis of SARS. J. Pathol. 210: 288-297.
73. Jia H (2016) Pulmonary angiotensin-converting enzyme 2 (ACE2) and inflammatory lung disease. Shock 46: 239-248.
74. Imai Y, Kuba K, Rao S, et al. (2005) Angiotensin-converting enzyme 2 protects from severe acute lung failure. Nature 436: 112-116.
75. Zhang R, Yu Y, Ni L, et al. (2020) COVID-19: melatonin as a potential adjuvant treatment. Life Sci. 250: 117583.
76. Anderson G, Reiter RJ. (2020) Melatonin: roles in influenza, COVID-19 and other viral infections. Rev. Med. Viral. 21: e2109.
77. Shneider A, Kudriavtsev A, Vakhrusheva A. (2020) Can melatonin reduce the severity of COVID-19 pandemic? Int. Rev. Immunol. 29: 1-10.
78. Reiter RJ, Abreu-Gonzalez P, Marik PE, et al. (2020) Therapeutic algorithm for the use of melatonin in patients with COVID-19. Front. Med. 7: 226.
79. Tain YL, Huang LT, Hsu CN, et al. (2014) Melatonin therapy prevents programmed hypertension and nitric oxide deficiency in offspring exposed to maternal calorie restriction. Oxid. Med. Cell. Longev. 2014: 283180.
80. Zou Z, Yun Y, Shu Y, et al. (2014) Angiotensin-converting enzyme 2 protects from lethal avian influenza A H5N1 infections. Nat. Commun. 5: 3594.
81. Gu H, Xie Z, Li T, et al. (2016) Angiotensin-converting enzyme 2 inhibits lung injury induced by respiratory syncytial virus. Sci. Rep. 6: 19840.
82. Abedi F, Hayes AW, Reiter R, et al. (2020) Acute lung injury: the therapeutic role of Rho kinase inhibitors. Pharmacol. Res. 155: 104736.
83. Borin TF, Arbab AS, Gelaleti GB, et al. (2016) Melatonin decreases breast cancer metastasis by modulating Rho-associated kinase protein-1 expression. J. Pineal Res. 60: 3-15.
84. Liu Z, Zou D, Yang X, et al. (2017) Melatonin inhibits colon cancer RKO cell migration by downregulating Rho-associated protein kinase expression via the p38/MAPK signaling pathway. Mol. Med. Rep. 16: 9383-9392.
85. Su SC, Hsieh MJ, Yang WE, et al. (2017) Cancer metastasis: mechanisms of inhibition by melatonin. J. Pineal Res. 62: 12370.
86. Boga JA, Coto-Montes A, Rosales-Corral SA et al. (2012) Beneficial actions of melatonin in the management of viral infections: a new use for this “molecular handyman”? Rev. Med. Virol. 22: 323-338.
87. Lu R, Liu Z, Shao Y, et al. (2019) Melatonin is responsible for rice resistance to rice stripe virus infection through a nitric oxide-dependent pathway. Virol. J. 16: 141.
88. Zhou Y, Hou Y, Shen J, et al. (2020) Network-based drug repurposing for novel coronavirus 2019-nCoV/SARS-CoV-2. Cell Discov. 6: 14.
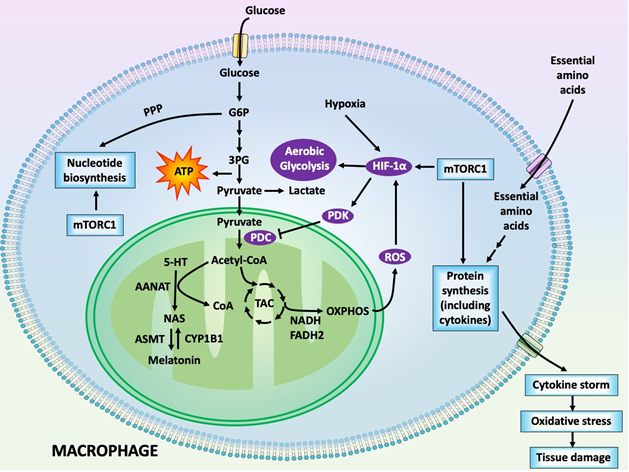

This work is licensed under a Creative Commons Attribution 4.0 International License.
For all articles published in Melatonin Res., copyright is retained by the authors. Articles are licensed under an open access Creative Commons CC BY 4.0 license, meaning that anyone may download and read the paper for free. In addition, the article may be reused and quoted provided that the original published version is cited. These conditions allow for maximum use and exposure of the work, while ensuring that the authors receive proper credit.
In exceptional circumstances articles may be licensed differently. If you have specific condition (such as one linked to funding) that does not allow this license, please mention this to the editorial office of the journal at submission. Exceptions will be granted at the discretion of the publisher.