Melatonin and the Optics of the Human Body
Abstract
Melatonin is fundamental to the lighting, display, and architectural industries as the primary biomarker used in circadian theory. Billions of dollars are being spent on research, product development, and marketing based on the impact of visible light on melatonin produced by the pineal gland. It has now been shown that the mitochondria produce melatonin in many cells in quantities which are orders of magnitude higher than that produced in the pineal gland. This subcellular melatonin does not necessarily fluctuate with our circadian clock or release into the circulation system, but instead has been proposed to be consumed locally in response to the free radical density within each cell, in particular in response to Near Infrared (NIR) exposure. The main point of this review hypothesizes that the subcellular melatonin is being produced in response to the NIR photons which make up the majority of natural sunlight. Given the number of cells and quantity of subcellular melatonin identified to date, it is reasonable to propose that the body produces and maintains a melatonin reservoir that is separate and apart from the circulatory melatonin generated by the pineal gland. To understand how sunlight may support or stimulate this antioxidant reservoir, it becomes necessary to quantify the free radical density in various parts of the human body. To do this, it is necessary to move away from two-dimensional empirical approaches and develop three-dimensional bio-optical models based on the underlying biological processes at play. Three-dimensional Mechanistic Bio-optical Models (MBM) of the skin, eye, and brain based on non-sequential optical ray tracing and Electron Spin Resonance (ESR) data clearly indicate that the NIR portion of natural sunlight provides the primary stimulus during the day to the majority of the cells in the human body, impacting over 60% of the cells in an adult body and 100% of the cells in the fetus and young children. It is also shown that optically, the human body, under the assumption of natural sunlight, has developed optical mechanisms to gather and localize NIR photons in the most sensitive areas of the human body: blood vessels, retina, brain, skin, and even the fetus. That assumption is no longer valid in modern societies where the majority of our time is spent exposed to visible only lighting and displays, which emit zero NIR photons. Based on an optical and biological review of the literature and the MBM results, it is proposed that the NIR portion of natural sunlight stimulates an excess of antioxidants in each of our healthy cells and that the cumulative effect of this antioxidant reservoir is to enhance the body’s ability to rapidly and locally deal with changing conditions throughout the day. In this approach the role of circulatory melatonin produced by the pineal gland is to provide an efficient method of delivering supplemental melatonin during periods of low cellular activity and solar stimulus to damaged or aging cells in both diurnal and nocturnal animals. While circulatory melatonin may be the “Hormone of Darkness”, subcellular melatonin may be the “Hormone of Daylight”.
References
2. Suofu Y, et al. (2017) Dual role of mitochondria in producing melatonin and driving GPCR signaling to block cytochrome c release. PNAS 114 (38): E7997-E8006.
3. He C, Wang J, Zhang Z, Yang M, Li Y, Tian X, Ma T, Tao J, Zhu K, Song Y, Ji P, Liu G (2016). Mitochondria Synthesize Melatonin to Ameliorate Its Function and Improve Mice Oocyte's Quality under in Vitro Conditions. Int. J. Mol. Sci. 17 (6): E939. doi: 10.3390/ijms17060939.
4. Wang L, Feng C, Zheng X, Guo Y, Zhou F, Shan D, Liu X, Kong J. (2017) Plant mitochondria synthesize melatonin and enhance the tolerance of plants to drought stress. J. Pineal Res. 63(3): E12429. doi: 10.1111/jpi.
5. Odinokov D, Hamblin MR (2018) Aging of lymphoid organs: Can photobiomodulation reverse age-associated thymic involution via stimulation of extrapineal melatonin synthesis and bone marrow stem cells? J. Biophot. 11 (8): e201700282.
6. Dimakatso M et al (2018) Role of photobiomodulation on the activation of the smad pathway via TGF-β in wound healing. J. Photochem. Photobio. B: Bio. 189: 138-144
7. Hamblin M (2016) Shining light on the head: photobiomodulation for brain disorders. BBA Clinical 6: 113-124.
8. Merry GF, et al (2017) Photobiomodulation reduces drusen volume and improves visual acuity and contrast sensitivity in dry age-related macular degeneration Acta Ophthalmol. 95 (4): e270-e277
9. Cassano P, et al (2018) Transcranial photobiomodulation for the treatment of major depressive disorder, the elated-2 pilot trial. Photomed. Laser Surg. doi: 10.1089/pho.2018.4490.
10. Reiter RJ, et al. (2017) Melatonin as a mitochondria-targeted antioxidant: one of evolutions best ideas. Cell Mol. Life Sci. 74: 1863-1881
11. Bonnefort-Rousselot D, Collin F (2010) Melatonin: Action as antioxidant and potential applications in human disease and aging. Toxicology 278 (1):55-67.
12. Slominski A, et al. (2018) Melatonin: A cutaneous perspective on its production, metabolism, and functions. J. Invest. Derm. 138 (3): 490-499.
13. Blasiak J, et al. (2016) Melatonin in retinal physiology and pathology: The case of age-related macular degeneration. Oxid. Med. Cell Longev. 2016: 6819736
14. Acuna-Castroviejo D, et al. (2014) Extrapineal melatonin: sources, regulation, and potential functions. Cell Mol. Life Sci. 71 (16): 2997-3025. doi: 10.1007/s00018-014-1579-2.
15. Koltover V (2017) Free radical timer of aging: from chemistry of free radicals to systems theory of reliability. Curr. Aging Sci. 10 (1): 12-17.
16. Zhao J, et al. (2012) Red light and the sleep quality and endurance performance of chinese female basketball players. J. Athletic. Train. 47 (6) : 673-678.
17. Kumar J, et al. (2015) Circadian rhythms in glucose and lipid metabolism in nocturnal and diurnal mammals. Mol. Cell Endocrinol. 418: Pt 1:74-88.
18. Kumar H, et al. (2012) the role of free radicals in the aging brain and the parkinson’s disease: convergence and parallelism. Int. J. Mol. Sci. 13 (8): 10478-10504.
19. Bashkatov A, et al. (2006) Optical properties of human cranial bone in the spectral range from 800 to 2000nm. Proc. of SPIE 6163: 616310-9
20. Borjigin J, et al. (2012) Circadian regulation of pineal gland rhythmicity. Mol. Cell Endocrinol. 349 (1): 13-19.
21. Tirpak A, Young R (2008) Accurate transmission measurements of translucent materials. Photonics Spectra https://www.photonics.com/Article.aspx?AID=32297.
22. Shishegar N, Boubekri M (2016) Natural light and productivity: analyzing the impacts of daylighting on students’ and workers’ health and alertness. Conference: Int. Conf. on “Health, Biological and Life Science” (HBLS-16), at Istanbul, Turkey.
23. Anders J. (2017) Photobiomodulation therapy comes of age. BioPhotonics 24(2): 28
24. Bianconi E et al. (2013) An estimation of the number of cells in the human body. Ann. Hum. Biol. 40 (5): 463-471.
25. Kuboyama N, Abiko Y (2012) Reduction of monocyte chemoattractant protein-1 expression in rheumatoid arthritis rat joints with light emitting diode. Laser Ther. 21 (3): 177-181.
26. Abdallah M, et al. (2012) Amniotic fluid chemokines and autism spectrum disorders: An exploratory study utilizing danish historic birth cohort. Brain Behav. Immun. 26 (1): 170-176.
27. Yennu A, et al. (2016) Prefrontal responses to stroop tasks in subjects with post-traumatic stress disorder assessed by functional near infrared spectroscopy. Sci. Rep. 6: 30157. doi: 10.1038/srep30157
28. Zastrow L, et al. (2009) Detection and identification of free radicals by UV and visible light in ex vivo human skin. Intl. J. Cos. Sci. 31 (5):207-215.
29. Guy G, et al. (2015) Vital Signs: Melanoma incidence and mortality trends and projections – united states 1982 to 2030. MMWR June 2015
30. Zastrow L, et al. (2015) Free radical threshold value: A new universal body constant. Skin Pharm. Phys. 28 (5): 264-268.
31. Haywood R, et al. (2007) Protein, lipid, and DNA radicals to measure skin UVA damage and modulation by melanin. Free Rad. Bio. Med. 44 (6): 990-1000.
32. Zastrow L, et al. (2015) Free radical threshold value: A new universal body constant. Skin Pharm. Phys. 28 (5):264-268.
33. Hamblin M, et al. (2018) Low-level light therapy: Photobiomodulation. SPIE Press Book
34. Keszler A, et al. (2017) Red/near Infrared light stimulates release of an endothelium dependent vasodilator and rescues vascular dysfunction in diabetes model. Free Rad. Biol. Med. 113: 157-164. doi: 10.1016/j.freeradbiomed.2017.09.012.
35. Wang X, et al. (2016) Interplay between up-regulation of cytochrome-c-oxidase and hemoglobin oxygenation induced by near-infrared laser. Sci. Rep. 6: 30540.
36. Adolescent Brain Cognitive Development Study. https://abcdstudy.org
37. Yacan S. (2014) Impact of daylighting on preschool students’ social and cognitive skills. Thesis U of Nebraska 2014.
38. Lockley S, et al. (2003) High sensitivity of the human circadian melatonin rhythm to resetting by short wavelength light. J. Clin. Endocrinol. Metab. 88 (9): 4502-4505
39. Figueiro M, Rea M (2010) The effects of red and blue lights on circadian variations in cortisol, alpha amylase, and melatonin. Int. J. Endocrinol. 2010: 829351. doi: 10.1155/2010/829351.
40. Son J, et al. (2017) A novel combination treatment to stimulate bone healing and regenerate under hypoxic conditions: Photobiomodulation and melatonin. Lasers Med. Sci. 32 (3): 533541.
41. Abbaszadeh A, et al. (2017) Melatonin role in ameliorating radiation-induced skin damage: From theory to practice (a review of literature). J. Biomed. Phys. Eng. 7: 127-136.
42. Popovic B, et al. (2018) The influence of ageing on the extrapineal melatonin synthetic pathway. Exp. Gerontol. 110: 151-157.
43. Odinokov D, Hambiln MR (2018) Aging of lymphoid organs: Can photobiomodulation reverse age-associated thymic involution via stimulation of extrapineal melatonin synthesis and bone marrow stem cells? J. Biophot. 11 (8): e201700282.
44. Slominski AT, et al Melatonin, mitochondria, and the skin. Cell Mol. Life Sci. 74 (21): 39133925.
45. Barolet D, et al. (2016) Infrared and skin: friend or foe. J. Photochem. Photobio. B: Bio. 155 : 78-85
46. Kim TK, et al. (2015) Melatonin and its metabolites accumulate in the human epidermis in vivo and inhibit proliferation and tyrosinase activity in epidermal melanocytes in vitro. Mol. Cell Endocrinol. 404: 1-6.
47. Barolet D, Boucher A (2008) LED photoprevention: reduced MED response following multiple LED exposures. Lasers Surg. Med. 40 (2): 106-112.
48. Skobowiat C, et al. (2018) Melatonin and its derivatives counteract the ultraviolet B radiation induced damage in human and porcine skin ex vivo. J. Pineal Res. 65: e12501. doi: 10.1111/jpi.12501.
49. Hill SM, et al. (2015) Melatonin: An inhibitor of breast cancer. Endocr. Relat. Cancer 22 (3): R183-R204.
50. Zhang Y, et al. (2013) Visible and near-Infrared spectroscopy for distinguishing malignant tumor tissue from benign tumor and normal breast tissues in vitro. J. Biomed. Optics 18 (7): 077003.
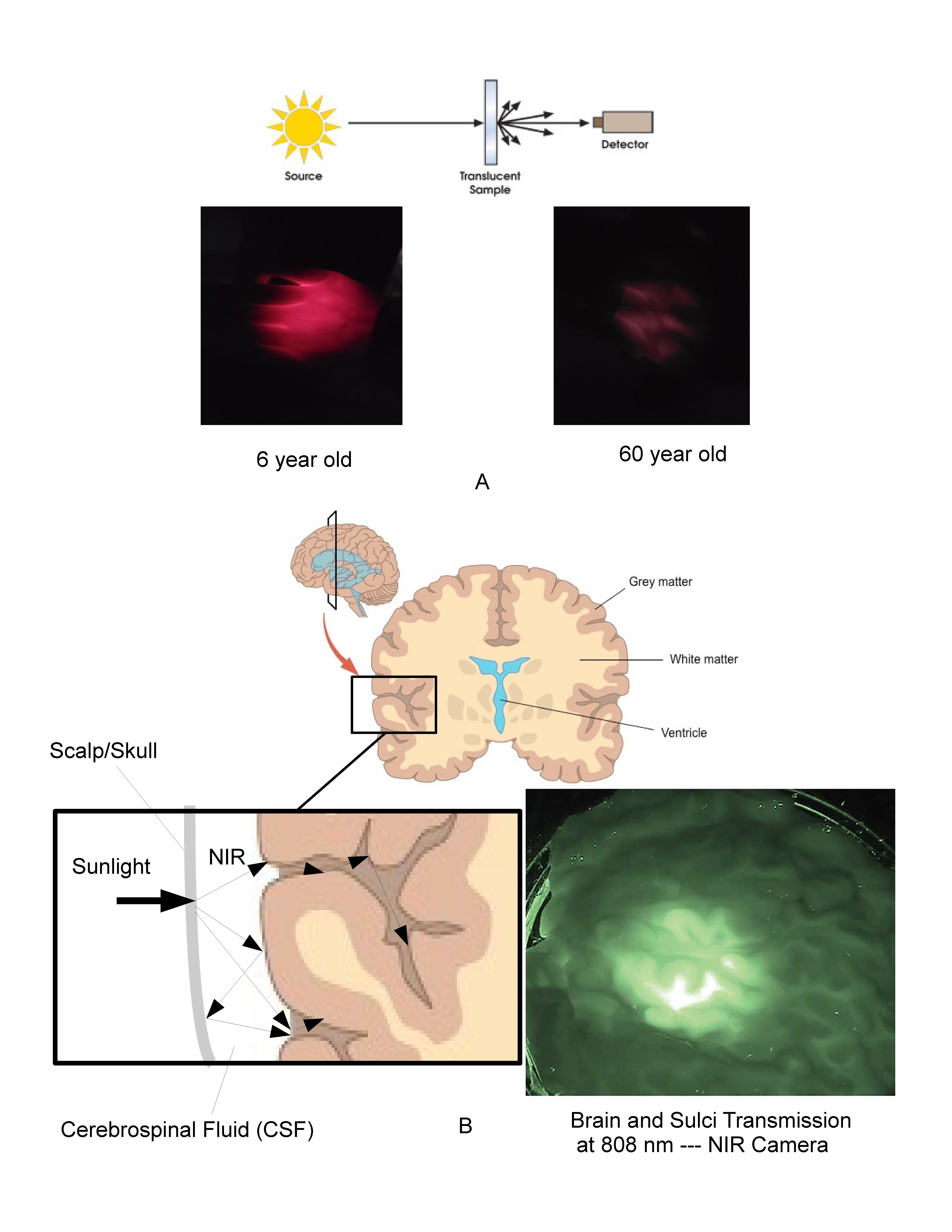

This work is licensed under a Creative Commons Attribution 4.0 International License.
For all articles published in Melatonin Res., copyright is retained by the authors. Articles are licensed under an open access Creative Commons CC BY 4.0 license, meaning that anyone may download and read the paper for free. In addition, the article may be reused and quoted provided that the original published version is cited. These conditions allow for maximum use and exposure of the work, while ensuring that the authors receive proper credit.
In exceptional circumstances articles may be licensed differently. If you have specific condition (such as one linked to funding) that does not allow this license, please mention this to the editorial office of the journal at submission. Exceptions will be granted at the discretion of the publisher.